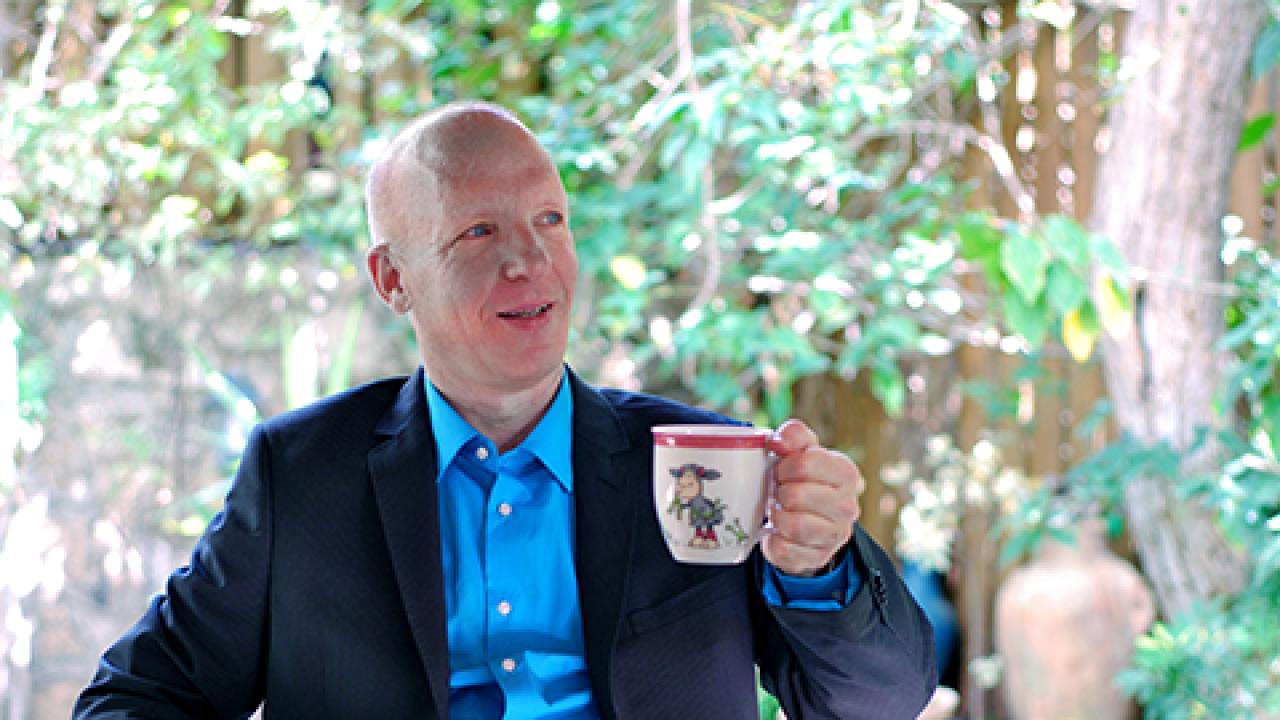
Yuval Gefen is one of the founding fathers of mesoscopic physics. One of his predictions—that currents traveling through extremely small pieces of metal or semiconductor material can be measured in single electrons—helped establish the lower limit of what characterizes the flow of electricity, and eventually led to the experimental design of a single-electron transistor. Gefen visited ICTP in February to give a Colloquium on "Weak Measurement: A Peephole into the Quantum World".
In the academic genealogy of physics, Gefen, who holds the Isabelle and Samuel Friedman Professorial Chair of Theoretical Physics at the Weizmann Institute, is the scientific great-grandchild of ICTP founder Abdus Salam: one of Gefen’s PhD advisors, Amnon Aharony, was a PhD student of the late Yuval Ne'eman, who was a student of Salam’s. While he was in Trieste, Gefen granted an interview to share his views on a wide range of topics, from entanglement to philosophy to quantum computers.
The conversation began with a reference to Lord Kelvin’s famous “clouds” speech ("Nineteenth-Century Clouds over the Dynamical Theory of Heat and Light”); Lord Kelvin’s minor “clouds” ignited a revolution in physics that brought about modern physics and the development of quantum and relativity theory. We started Gefen’s interview by asking him what he thought were some of the "clouds” of the 21st century in modern condensed matter physics. How will they influence new directions in the field?
Gefen: The two main challenges now are rooted in the physics of the early 20th century, but they didn’t get the attention they deserved. The first one has to do with entanglement, a major pillar of quantum mechanics. Quantum mechanics, against all our instincts and intuition, is non-local. If we accept this premise, then everything is entangled with everything. Then, the question is, how can we in physics talk about two separate systems? We would like to assume that you and me are two separate entities, but quantum mechanics paradoxically will tell us that we are not. How come this concept of entanglement could eventually lead to separation of entities? Why can we separate a detector from a system and read off the results of a detector? The notion of the loss of entanglement on a certain scale, the separability of things, is something that is very poorly understood.
The second issue is something that was tackled by giants of the 20th century, including Schrodinger and Heisenberg, and this has to do with the attempt to describe consciousness in terms of physics. Let me give you two examples: I perform a certain measurement: I have a system, and I have a detector that measures the system. This much I can describe by the equations of physics. But now, I take the detector and read off the screen, and there is some number that goes into my consciousness, and when I say “It is 20.5”, what does it mean? What is this process that fixes this number? In the jargon, one calls it “collapse of the system”: the system in principle could have been 20 or 21, there could have been many results of the experiments. But at the end of the day the measuring device and my consciousness decided the value of the system was 20.5: the system has “collapsed” into this value. What is this mysterious collapse? That is something that is not understood. What is the interaction between our consciousness and the actual detection of things?
As a follow up on this idea, I note that the great minds I mentioned were bothered a lot by the issue of determinism and free will. What is this interface between the consciousness and the reality which is described by physics equations?
ICTP: The 2016 Nobel Prize honoured three scientists for their theoretical discoveries of topological phase transitions and topological phases of matter. What is the role of topology in modern condensed matter physics?
Gefen: Let me project something very personal about that. I did my second postdoc with David Thouless (who won half of the 2016 Nobel Prize) and we have since remained in close touch. He was a mentor not only in physics: in many ways he taught me how to think, and how to integrate into the world of physics many other facets of life. He has fantastic insights and views concerning culture, literature, history, you name it. I recall sitting with him and enjoying every moment.
To put topology into perspective, we should go back more than 100 years. There have been several revolutions in our understanding of the material world. Going back to the late 19th century, people realized there were chemical elements and you can make compounds of those elements. Chemistry was dealing with the properties of materials given their composition.
Now, fast-forward to the mid 20th century, people realized that the chemical composition of materials was not sufficient, one needs to understand how the molecules are arranged, and that led to the concept of symmetry. Symmetry invaded all areas of physics. In solid state physics, it played an extremely important role; people understood that the optical properties of materials, their electrical properties, their magnetic properties, are all affected by symmetry. If we can influence material such that its symmetry is abruptly changed, we say that this material undergoes phase transition. Phase transitions have become a major theme in physics.
Now we fast-forward again to 50 years later, about 12 years ago, and there is an increasingly ubiquitous realization that symmetry is not sufficient. Topology undermining the structure of materials is the new concept, the new revolution. Topology, contrary to symmetry, is a concept which is non-local: I can take a material and try to influence it locally, and this will not affect its topology. The fact that topology is concerned with non-local properties introduced the idea that maybe there are properties that are really robust, protected against small, undesired bad influences. That led us in two directions. From the fundamental point of view, we are witnessing the ongoing revolution of topology; from the perspective of potential applications, people strongly believe that this robustness may be harnessed to design so-called quantum computers or quantum information processing devices. Just two years ago, I would have told you that it is an amazingly interesting concept, but as far as talking about quantum computing and information processing I would have said it looks sort of far fetched. But things are changing fast. There have been very specific, concrete proposals for experimental realization of quantum information devices; development of these is now underway. People are seriously talking about having some prototypes of quantum information devices—very primitive prototypes, but still, concrete devices. It is not science fiction anymore, it is a real achievable science.
--Mary Ann Williams